Research Highlights
Mechanics and thermodynamics of (Mg,Fe)O grain boundaries in planetary interiors (April 26,2024)

fig
A collaborative team of researchers from the Geodynamics Research Center, Ehime University and the Department of Earth Sciences, Utrecht University applied massive parallel high-performance computer simulations based on first principles quantum mechanical modeling to shed new light on the enigmatic behavior of grain boundaries under extreme pressures that prevail in planetary interiors. In particular, the mechanical behavior and thermodynamic properties of high-angle tilt grain boundaries in (Mg,Fe)O ferropericlase, the second most abundant mineral in the Earth’s lower mantle and possibly in the mantles of super-Earth exoplanets, were examined. In this study, in addition the standard density functional theory, the internally consistent LDA+U method was applied to reproduce the electronic structure of iron more accurately.
Their results on the mechanical behavior indicate that the very high pressure conditions in terrestrial planets have a strong effect on the mechanisms of grain boundary motion which govern intercrystalline deformation (figure). This research proved for the first time that structural transformations of grain interfaces, induced by pressure with increasing depth in planetary mantles, are able to trigger a change in the mechanism and in the direction of grain boundary motion. The results further demonstrate that significant mechanical weakening of grain boundaries can develop under multi-megabar pressures. This is counterintuitive because it is usually thought that with increasing pressure, atomic arrangements in materials become more closely packed and then materials get harder. Dr. This phenomenon of grain boundary weakening is caused by a change in the transition state structure of grain boundaries during their motion under extremely high pressures. Analyses of the data identify grain boundary weakening in ferropericlase as one of the potential mechanisms for viscosity reductions with increasing depth in the mantle of super-Earth exoplanets.
Additional thermodynamic modeling of the iron partitioning behavior between bulk and grain boundaries was then performed. It was elucidated that grain size is an important factor to control grain boundary segregation of iron in polycrystalline ferropericlase in the hot and dense lower mantle. It is well known that incorporation of substitutional Fe(II) in bulk MgO has a significant effect on its physical properties such as density and seismic wave velocities, since Fe(II) undergoes an electronic spin transition at high pressure in the Earth’s interior. Although there has been no information about the spin states of Fe(II) within grain boundaries, the present modeling shows that the electronic spin state of Fe(II) within ferropericlase tilt grain boundaries is controlled by structural grain boundary transformations at high pressure in Earth’s lower mantle. This mechanism influences the pressure condition of the iron spin crossover in polycrystalline (Mg,Fe)O with micrometer or smaller grain sizes. These findings indicate that the iron spin crossover pressure in ferropericlase may increase by several tens of GPa due to pressure-induced structural grain boundary transitions in dynamically active fine-grained lower mantle regions compared to more thermodynamically stable regions in the lower mantle.
Although several novel findings have been earned, more systematic data from theoretical modeling as well as from experiments and electron microscopy observations will be needed in future research to achieve better insights in the collective effects of grain boundaries on the rheological and thermodynamic properties of polycrystalline ferropericlase at the appropriate pressures and temperatures in planetary mantles.
S. Ritterbex, T. Tsuchiya, M. Drury, and O. Plumper, Atomic-scale study of intercrystalline (Mg,Fe)O in planetary mantles: Mechanics and thermodynamics of grain boundaries under pressure. Journal of Geophysical Research: Solid Earth 129, 5, e2023JB028375, 2024, DOI:10.1029/2023JB028375
Lattice thermal conductivity of lower mantle minerals from ab initio mineral physics (March 30, 2024)

fig.1
Lattice thermal conductivity, Klat, is a fundamental physical parameter in controlling the activity of heat transfer in a solid. It attracts researchers from wide ranging fields such as materials science, engineering, and Earth and planetary science. The determination of Klat of Earth’s constituent materials is key to understanding the dynamics and thermal evolution of the Earth’s interior. Despite its importance, studies on the measurement of Klat of the Earth’s lower mantle (LM) minerals at high pressure (P) and temperature (T) have, thus far, been limited; most likely due to the technical difficulties involved in carrying out these experiments. Therefore, extrapolation of Klat measured at limited P and/or T conditions is often made to discuss the thermal transport properties of the Earth’s LM.
Prof. Taku Tsuchiya and I have been conducting computational determinations for Klat of the LM minerals at any P and T conditions. Our approach is based on the density functional theory (DFT) combining with phonon transport theory describing anharmonic phonon-phonon interaction. In the last decade, we successfully determined the Klat of the end-members of the LM minerals, MgO periclase (Pe), MgSiO3 bridgmanite (Brg) and post-perovskite (PPv) under deep mantle P and T conditions (Dekura et al., 2013; Dekura & Tscuchiya, 2017; 2019) (Fig. 1). The relation of Klat of the LM end-member phases is found to be Klat^MgO>>Klat^PPv>Klat^(BrgKlat ) The significantly high Klat of MgO can be explained by large phonon lifetime of MgO, which is more than twice larger than that of the PPv and Brg. This is because the number of atoms in the primitive unit cell is only two with six phonon branches, resulting in weaker phonon-phonon scattering.

fig.2
Next, we recently extended our computational techniques to Fe-bearing systems, more realistic composition in the LM, in conjunction with the internally consistent DFT+U method (Wang et al., 2015) to deal with the electronic structures of such strongly correlated systems. The method was then applied to the Fe-bearing (Mg,Fe)O ferropericlase, the second most abundant LM mineral, and strong negative iron effect on the Klat of MgO was predicted (Dekura & Tsuchiya, 2023). For example, the Klat of MgO, which is ~20 and ~80 Wm-1K-1 at 30 and 136 GPa at 2000 K, respectively, is substantially reduced to be ~3 and ~30 Wm-1K-1 by the 12.5 mol% FeO incorporation (Fig. 2). We elucidated the physical origin of the observed strong negative iron effects on the Klat of MgO. Our analysis demonstrates that the strong iron effect is caused by decrease in both anharmonic phonon lifetime and phonon group velocity by the iron incorporation. The decrease in the phonon group velocity is due to the replacement of Mg atoms with Fe atoms of larger mass, and the decrease in the lifetime is related to the larger number of anharmonic decay channels for the three-phonon process.
It is widely accepted that the major mineral components of the LM are thought to be (Mg,Fe)SiO3 Brg in the most part and its high-P phase, PPv in the base. The Klat of these phases, therefore, primally controls the LM conductivity. We are currently investigating the effect of iron solid solution on both phases to provide a realistic thermal transport model of the lowermost mantle.(Haruhiko Dekura)
Nitrogen metal/silicate partitioning and implications for Earth’s missing nitrogen problem
(June 30, 2023)
Nitrogen (N) is one of the most significant volatiles in our planet, an essential element for life, and the primary component of the atmosphere. Our bulk silicate Earth (BSE) is depleted by an order of magnitude in N compared with other volatiles, such as carbon (C), water and noble gases, which is considered as the missing N problem of the BSE. This missing N problem is also manifested by the super-chondritic C/N ratio of the BSE. Several hypotheses have been proposed to account for this anomalous depletion. Different models proposed to explain the N deficit of the BSE are caused primarily by the difference in the measured partition coefficients of N between Fe and silicate melts (denoted by DN) at high pressure-temperature conditions. To clarify the partitioning behavior of N at conditions relevant to the core-mantle differentiation, we performed ab initio molecular dynamics combined with the thermodynamic integration method to calculate DN to 135 GPa and 5000 K.
Our calculations predict DN to be 3 (for NH3 speciation)~32 (for N2 speciation) at 20 GPa, 109~209 at 60 GPa and 84~491 at 135 GPa (Figure). And DN increases to 4~54 as temperature decreases from 5000 K to 4000 K at 20 GPa. The calculated DN values at 20 GPa are in good agreement with previous studies. These results show opposite effects of pressure and temperature on DN. In particular, they suggest a nonlinear effect of pressure on metal/silicate partitioning of N. Specifically, N becomes much more siderophile from 20 GPa to 60 GPa. And the effect of pressure is less significant from 60 GPa to 135 GPa. We also evaluate the effect of the core composition on N partitioning. When 10 at% Fe is substituted by Sulfur(S), DN reduces from 491 to 213, indicating that the presence of S in the metallic melt decreases the siderophility of N. The incorporation of 10 at% Silicon(Si) into the metallic melt leads to a reduction of DN to 188. In contrast, Oxygen(O) has an opposite effect on DN in comparison with S and Si, where DN increases to 611. Overall, N is strongly siderophile at these conditions even though several weight percent light elements are present in the metallic melt.
The structural and electronic analysis is performed to reveal the mechanism of N partitioning. There is a significant N-N bond in the silicate melt for N2 speciation, and a N-H bond in the silicate melt for NH3 speciation. However, with increasing pressure, the coordination number (CoN) of N by N decreases from 0.87 to 0.49~0.42, whereas the CoN of N by Si increases from 0.37 at 20 GPa to 1.13 at 60 GPa and to 2.07 at 135 GPa. These results indicate that about half of molecular N dissociates and bonds with Si. Similarly, in NH3-bearing silicate melt, the CoN of N by Si increases with pressure. These results imply that N behaves as anion in the silicate melt and interacts more with the silicate framework upon compression, which is nearly independent of redox states. The nonlinear effect of pressure on DN is related to the structural changes of the silicate melt under high pressure. Such pressure-induced structural densification is likely to promote the dissociation of molecular N in the silicate melt, and control the partitioning behavior of N under high pressure.
Using our predicted partition coefficients, we model the distribution of N during deep core-mantle differentiation. The geochemical modeling results demonstrate that more than 70% N is stored in the metallic core after core-mantle separation and less than 10% N resides in the mantle. These imply that the Earth’s core is likely the largest reservoir of N, and the mantle can be depleted in N due to deep core-mantle differentiation. Our modeling further shows that at shallow magma ocean conditions, where DN is smaller than DC, the C/N ratio of the BSE decreases during core-mantle differentiation. Whereas at deep magma ocean conditions, this value increases because N becomes more siderophile than C at very high pressure. Therefore, fractionation between N and C at deep magma ocean can increase the C/N ratio to the value of the current BSE. (Shengxuan Huang, PD, JSPS, GRC)
Sound velocities in the deep lunar mantle (June 1, 2022)

fig.1
The Moon has undergone differentiation and is composed of a crust (35~45 km), a mantle, and a small metallic core (< 350 km), as shown in Figure 1c. The composition of the lunar interior is largely unconstrained; however, the deep lunar mantle is likely to be composed to olivine (ol), pyroxene (px), and garnet (gt) (Wieczorek+ Rev. Mineral Geochem. 2006; Taylor Geochim. Cosmochim. Acta 2014). Recent studies on the crystallisation of the lunar magma ocean (LMO) indicate that garnet may play a significant role in the deep lunar mantle (Jing+ Earth Planet. Sci. Lett. 2022; Kraettli+ Icarus 2022, Johnson+ Earth Planet. Sci. Lett. 2021, Elardo+ Geochim. Cosmochim. Acta 2011). Understanding the sound velocities of realistic lunar mantle aggregates is critical for the interpretation of lunar seismic velocity models, and constraints on the temperature and composition of the planetary interior.
Aggregates of lunar mantle composition, shown in Figure 1a and 1b, containing a significant proportion of garnet, alongside olivine and orthopyroxene, were synthesised from glasses at high pressure and temperature using the multi-anvil press. In situ experiments were carried out at BL04B1 at SPring-8 where combined measurements of ultrasonic interferometry, X-ray diffraction, and X-ray radiography were taken. These measurements were taken at a range of temperature and pressure conditions of relevance to the deep lunar interior.
The results are shown in Figure 2, where global fits to P-T-Vp,Vs are shown alongside lunar seismic velocity models from the literature. This study is indicated with the solid line and seismic velocity models from the literature are shown with dashed lines. Crosses indicate models at 300 K alongside the fitted data and seismic velocities; these are calculated using finite-strain EOS to determine the elastic moduli and densities of each mineral, combined with their phase proportions. These sound velocities in deep lunar mantle compositions allow us to draw compositional constraints on the lunar interior, and indicate the possible presence of garnet dependent on the lunar thermal profile. (Marisa Wood)
Crystal preferred orientation of phase D (October 30, 2022)

fig
Growing evidence suggests that near subducted slabs, the mantle transition zone and uppermost lower mantle are seismically anisotropic (Vsh > Vsv), especially near Tonga (Chen and Brudzinski, 2003; Ferreira et al., 2019; Lynner and Long, 2015; Nowacki et al., 2015). In view of the dynamic process of both the mantle and subducted slabs, it is believed that crystallographic preferred orientation (CPO) of minerals constituting mantle and slab probably accounts for this kind of observed seismic anisotropy in the deep mantle. In cold subducting slabs, antigorite will transform into a series of hydrous minerals so called dense hydrous magnesium silicate (DHMS), which could stably exist in cold subducting slabs where the geotherm are relatively low, playing an important role in water transport into deep mantle. Among these DHMSs, phase D can be probably stable at conditions of the lower part of transition zone and the upper part of lower mantle. Its layered crystal structure where MgO6 octahedron interlayered with SiO6 octahedron layers renders phase D an anisotropic mineral both in rheology and elasticity, making their properties have profound influence on dynamic of subduction zones. Therefore, phase D is expected to be a good candidate to shed some light on these phenomena owing to its relatively high shear wave anisotropy even at higher pressure up to 48 GPa (Mainprice et al., 2007; Tsuchiya and Tsuchiya, 2008).
Rosa et al. (2013) first reported that phase D can easily form CPO where (0001) planes perpendicular with first principal stress direction under the conditions up to 48 GPa and room temperature based on DAC apparatus. However, the influence of temperature and stain rate have not been taken into account. By using D111-type deformation apparatus, we have conducted uniaxial deformation on the synthesized Mg-phase D (Mg1.12Si1.97H1.87O6) under 20 GPa and 500~1000℃, with strain rate of 3.54 × 10-6~3.69 × 10-5 s-1, which is close to the condition of mantle transition zone.
The consequent CPO of the deformed samples were acquired using 2D-XRD method because the crystals of phase D are too fragile to form Kikuchi band after exposure of accelerated electron beam. The refined results show maximum of (0001) poles generally along the first principal stress direction, with various intensities which are proportional with strain (0.04–0.4), and the final CPO intensities also have the positive correlation with the increased temperature. However, no other type of CPO was observed, indicating basal slip is still dominant in the experimental conditions.
The calculated seismic anisotropy using elastic constants and CPO of Mg-phase D indicate that fast shear wave generally polarize in horizontal plane, which may have contribution to radial anisotropy of Vsh > Vsv. The shear wave anisotropy can be up to 2.4%, making the delay time to be 0.22 s if 50 km of phase D aggregate in thickness in slabs and surrounding hydrous peridotite was assumed. Hence, the phase D with CPO could possibly be a contributor to the observed radial anisotropy.(Wentian Wu:愛媛大博士後期課程2年)
Sound velocity of partially molten rocks (March 31, 2022)

fig.1
P- and S-wave velocities are unique tools which we have to explore the bulk chemical composition and structures of the inaccessible deep Earth, by comparing high pressure mineral physics data with seismological observations. Such approaches have been successful in interpreting the Earth’s interior (e.g. Irifune+ Nature 2008; Gréaux+ Nature 2019) and is now applied to Martian compositions to interpret InSight seismic data (Xu+ GRL 2021). However, despite the extensive seismic surveys and numerous laboratory studies, mineral physics data still cannot explain a number of seismic discontinuities and scatterings, widespread at multiple depths across the globe (Ballmer+ Nat. Geo. 2015, Waszek+ Nat. Comm. 2016). A few pioneering studies proposed to explain some of the discrepancies at the 410 km and 660 km depth discontinuities by the presence of partial melt (Schmandt et al., Science 2014; Chantel+ Sci. Adv. 2016; Manthilake+ Nat. Comm. 2017, Miner. 2021) but their results, due to the limited information on the rock velocities and the nature and distribution of melt heterogeneities, do not permit to establish physical models and hence it is still difficult to fully interpret seismic observations in terms of mantle composition, structure and dynamics.Our current research project aims at evaluating and quantifying P- and S-waves velocities of inhomogeneous rock aggregates under simultaneous high pressures and high temperatures. Because investigating sound velocities upon partial melting in silicate systems can be technically challenging, we chose to start this project with more simplistic approach and investigated the sound velocities of olivine + FeS aggregates, as an analog to the partially molten mantle rock. The ultrasonic experiments were carried out by means of in situ ultrasonic interferometry, X-ray radiography and X-ray diffraction techniques at high pressure and high temperature in the 1500-ton multianvil press apparatus SPEED1500 located at the beamline BL04B1. All measurements were conducted continuously, in one heating ramp, upon melting of FeS in the silicate rock
matrix by means of an automated flipping slit system and a time-resolved data acquisition software newly developed in collaboration with beamline scientists at BL04B1 (Kono+ High P. Res. 2021). Figure 1 shows an example of successive X-ray diffraction patterns collected at 15 GPa, upon melting of FeS, in wadsleyite + 5 wt.% FeS while Figure 2 shows the corresponding P- and S-wave travel time (tP and tS, thereafter) as a function of increasing temperature.
A parallel analysis of the X-ray diffraction data and travel time measurements permitted to clearly interpret the physical changes in the sample as temperature increased. The first travel time decreases at ~750℃ corresponded to the release of deviatoric stress, which is completed after heating for about 30 min, while the second tP an tS decrease at T > 800℃ corresponded to the gradual disappearance of olivine from the starting material. At T higher than 950℃, the sample consists of wadsleyite + FeS(solid), which is confirmed by XRD data and the tP and tS first plateau. At ~1025℃ , FeS started to melt, which is characterized by a gradual increase of tP and tS and the disappearance of FeS peaks as well as an increase of the XRD background. At T ~1200℃ both tP and tS reached a second plateau where they do not further increase with increasing T, hence suggesting FeS is completely molten. These preliminary results show that it is possible to quantify the effect of heterogeneities on the velocities of rock samples while differentiating solid and melt heterogeneities. We plan in the future to apply those techniques to the investigations of more inhomogeneous samples with varying silicate matrix components and melt composition and combine this information with spatial distribution and aspect ratio analyses of the melt heterogeneities. This new information will be used to establish physical models that can be applied for interpreting regions of partial melting in the Earth’s interior such as the top of the 410 and beneath the 660, as well as to confirm or disprove the proposed existence of partial melting at the core-mantle-boundary of the Moon (Weber+ Science 2011; Khan+ J. Geophys. Res. 2014), and Mars (Samuel+ J. Geophys. Res. 2021). (Steeve Gréaux)
Low Shear Velocity Zones (LSVZ) in the Martian Upper Mantle Highlighted by Sound Velocity Measurements (October 12, 2021)
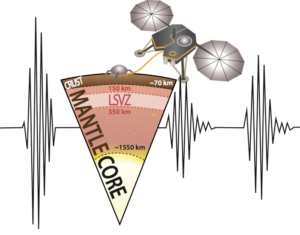
Schematic diagram of seismic low-velocity region existing in the upper mantle of Mars inferred from ultrasonic velocity measurements on Martian rock aggregates
A collaborative work conducted by researchers at the Geodynamics Research Center (GRC) and Institut de Minéralogie, de Physique des Matériaux et de Cosmochimie (IMPMC) suggest the existence of low shear seismic velocity regions between 150 and 350 km depth in Mars mantle. The experimental results presented in this study are consistent with recent observations from the InSight mission and could give important clues on Mars’ mantle mineralogy and internal structure.(Steeve Gréaux)
read more
The sound velocity of Majorite under mantle transition zone conditions (August 25, 2021)
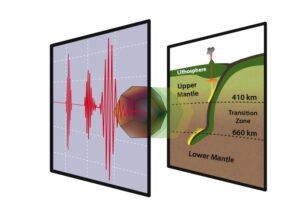
Schematic diagram of the subduction slab inferred from the ultrasonic velocity measurements on Majorite garnet
Researchers at Ehime University have reported the sound velocity of MgSiO3 Majorite garnet up to the pressure of 18 Gigapascals and temperature up to 2,000 Kelvins. Their results lead to understanding of the mineral composition of the Earth’s mantle transition zone (MTZ), which has not been fully elucidated yet. This study suggests that a mechanical mixture of slab and mantle rocks, rather than equilibrated rocks, is more likely to explain seismological observations throughout the MTZ.(Steeve Gréaux)
read more
Sound velocities in the deep lunar mantle (June 1, 2022)
The Moon has undergone differentiation and is composed of a crust (35~45 km), a mantle, and a small metallic core (< 350 km), as shown in Figure 1c. The composition of the lunar interior is largely unconstrained; however, the deep lunar mantle is likely to be composed to olivine (ol), pyroxene (px), and garnet (gt) (Wieczorek+ Rev. Mineral Geochem. 2006; Taylor Geochim. Cosmochim. Acta 2014). Recent studies on the crystallisation of the lunar magma ocean (LMO) indicate that garnet may play a significant role in the deep lunar mantle (Jing+ Earth Planet. Sci. Lett. 2022; Kraettli+ Icarus 2022, Johnson+ Earth Planet. Sci. Lett. 2021, Elardo+ Geochim. Cosmochim. Acta 2011). Understanding the sound velocities of realistic lunar mantle aggregates is critical for the interpretation of lunar seismic velocity models, and constraints on the temperature and composition of the planetary interior.
Aggregates of lunar mantle composition, shown in Figure 1a and 1b, containing a significant proportion of garnet, alongside olivine and orthopyroxene, were synthesised from glasses at high pressure and temperature using the multi-anvil press. In situ experiments were carried out at BL04B1 at SPring-8 where combined measurements of ultrasonic interferometry, X-ray diffraction, and X-ray radiography were taken. These measurements were taken at a range of temperature and pressure conditions of relevance to the deep lunar interior.
The results are shown in Figure 2, where global fits to P-T-Vp,Vs are shown alongside lunar seismic velocity models from the literature. This study is indicated with the solid line and seismic velocity models from the literature are shown with dashed lines. Crosses indicate models at 300 K alongside the fitted data and seismic velocities; these are calculated using finite-strain EOS to determine the elastic moduli and densities of each mineral, combined with their phase proportions. These sound velocities in deep lunar mantle compositions allow us to draw compositional constraints on the lunar interior, and indicate the possible presence of garnet dependent on the lunar thermal profile. (Marisa Wood)
Crystal preferred orientation of phase D (June 1, 2022)

fig
Growing evidence suggests that near subducted slabs, the mantle transition zone and uppermost lower mantle are seismically anisotropic (Vsh > Vsv), especially near Tonga (Chen and Brudzinski, 2003; Ferreira et al., 2019; Lynner and Long, 2015; Nowacki et al., 2015). In view of the dynamic process of both the mantle and subducted slabs, it is believed that crystallographic preferred orientation (CPO) of minerals constituting mantle and slab probably accounts for this kind of observed seismic anisotropy in the deep mantle. In cold subducting slabs, antigorite will transform into a series of hydrous minerals so called dense hydrous magnesium silicate (DHMS), which could stably exist in cold subducting slabs where the geotherm are relatively low, playing an important role in water transport into deep mantle. Among these DHMSs, phase D can be probably stable at conditions of the lower part of transition zone and the upper part of lower mantle. Its layered crystal structure where MgO6 octahedron interlayered with SiO6 octahedron layers renders phase D an anisotropic mineral both in rheology and elasticity, making their properties have profound influence on dynamic of subduction zones. Therefore, phase D is expected to be a good candidate to shed some light on these phenomena owing to its relatively high shear wave anisotropy even at higher pressure up to 48 GPa (Mainprice et al., 2007; Tsuchiya and Tsuchiya, 2008).
Rosa et al. (2013) first reported that phase D can easily form CPO where (0001) planes perpendicular with first principal stress direction under the conditions up to 48 GPa and room temperature based on DAC apparatus. However, the influence of temperature and stain rate have not been taken into account. By using D111-type deformation apparatus, we have conducted uniaxial deformation on the synthesized Mg-phase D (Mg1.12Si1.97H1.87O6) under 20 GPa and 500~1000℃, with strain rate of 3.54 × 10-6~3.69 × 10-5 s-1, which is close to the condition of mantle transition zone.
The consequent CPO of the deformed samples were acquired using 2D-XRD method because the crystals of phase D are too fragile to form Kikuchi band after exposure of accelerated electron beam. The refined results show maximum of (0001) poles generally along the first principal stress direction, with various intensities which are proportional with strain (0.04–0.4), and the final CPO intensities also have the positive correlation with the increased temperature. However, no other type of CPO was observed, indicating basal slip is still dominant in the experimental conditions.
The calculated seismic anisotropy using elastic constants and CPO of Mg-phase D indicate that fast shear wave generally polarize in horizontal plane, which may have contribution to radial anisotropy of Vsh > Vsv. The shear wave anisotropy can be up to 2.4%, making the delay time to be 0.22 s if 50 km of phase D aggregate in thickness in slabs and surrounding hydrous peridotite was assumed. Hence, the phase D with CPO could possibly be a contributor to the observed radial anisotropy.(Wentian Wu:愛媛大博士後期課程2年)
Sound velocity of partially molten rocks (October 30, 2022)

fig.1
P- and S-wave velocities are unique tools which we have to explore the bulk chemical composition and structures of the inaccessible deep Earth, by comparing high pressure mineral physics data with seismological observations. Such approaches have been successful in interpreting the Earth’s interior (e.g. Irifune+ Nature 2008; Gréaux+ Nature 2019) and is now applied to Martian compositions to interpret InSight seismic data (Xu+ GRL 2021). However, despite the extensive seismic surveys and numerous laboratory studies, mineral physics data still cannot explain a number of seismic discontinuities and scatterings, widespread at multiple depths across the globe (Ballmer+ Nat. Geo. 2015, Waszek+ Nat. Comm. 2016). A few pioneering studies proposed to explain some of the discrepancies at the 410 km and 660 km depth discontinuities by the presence of partial melt (Schmandt et al., Science 2014; Chantel+ Sci. Adv. 2016; Manthilake+ Nat. Comm. 2017, Miner. 2021) but their results, due to the limited information on the rock velocities and the nature and distribution of melt heterogeneities, do not permit to establish physical models and hence it is still difficult to fully interpret seismic observations in terms of mantle composition, structure and dynamics.

fig.2
Our current research project aims at evaluating and quantifying P- and S-waves velocities of inhomogeneous rock aggregates under simultaneous high pressures and high temperatures. Because investigating sound velocities upon partial melting in silicate systems can be technically challenging, we chose to start this project with more simplistic approach and investigated the sound velocities of olivine + FeS aggregates, as an analog to the partially molten mantle rock. The ultrasonic experiments were carried out by means of in situ ultrasonic interferometry, X-ray radiography and X-ray diffraction techniques at high pressure and high temperature in the 1500-ton multianvil press apparatus SPEED1500 located at the beamline BL04B1. All measurements were conducted continuously, in one heating ramp, upon melting of FeS in the silicate rock
matrix by means of an automated flipping slit system and a time-resolved data acquisition software newly developed in collaboration with beamline scientists at BL04B1 (Kono+ High P. Res. 2021). Figure 1 shows an example of successive X-ray diffraction patterns collected at 15 GPa, upon melting of FeS, in wadsleyite + 5 wt.% FeS while Figure 2 shows the corresponding P- and S-wave travel time (tP and tS, thereafter) as a function of increasing temperature.

fig.2
A parallel analysis of the X-ray diffraction data and travel time measurements permitted to clearly interpret the physical changes in the sample as temperature increased. The first travel time decreases at ~750℃ corresponded to the release of deviatoric stress, which is completed after heating for about 30 min, while the second tP an tS decrease at T > 800℃ corresponded to the gradual disappearance of olivine from the starting material. At T higher than 950℃, the sample consists of wadsleyite + FeS(solid), which is confirmed by XRD data and the tP and tS first plateau. At ~1025℃ , FeS started to melt, which is characterized by a gradual increase of tP and tS and the disappearance of FeS peaks as well as an increase of the XRD background. At T ~1200℃ both tP and tS reached a second plateau where they do not further increase with increasing T, hence suggesting FeS is completely molten. These preliminary results show that it is possible to quantify the effect of heterogeneities on the velocities of rock samples while differentiating solid and melt heterogeneities. We plan in the future to apply those techniques to the investigations of more inhomogeneous samples with varying silicate matrix components and melt composition and combine this information with spatial distribution and aspect ratio analyses of the melt heterogeneities. This new information will be used to establish physical models that can be applied for interpreting regions of partial melting in the Earth’s interior such as the top of the 410 and beneath the 660, as well as to confirm or disprove the proposed existence of partial melting at the core-mantle-boundary of the Moon (Weber+ Science 2011; Khan+ J. Geophys. Res. 2014), and Mars (Samuel+ J. Geophys. Res. 2021). (Steeve Gréaux)
How were the carbon contents in terrestrial and lunar mantles established? (June 1, 2021)
Carbon degassed from planetary mantles by volcanic activity plays an important role in the planetary surface environment. However, how the carbon content in the Earth’s mantle was established is still poorly understood. Here we show that the mantle of planetary embryos may have been nearly saturated with carbon by new high-pressure experiments and pointed out that the carbon solubility of magma is very consistent with the estimated carbon contents in terrestrial and lunar mantles. (Hideharu Kuwahara)
read more
A deep reservoir of primordial helium in the Earth (May 2, 2021)
A stable isotope of helium, 3He, was produced by the Big Bang and a remnant of the solar nebula.
3He is included in ocean island basalts, such as in Hawaiian volcanos, indicating it has been stored somewhere in the deep Earth and carried up to the surface in upwelling plumes.
New computer simulations demonstrate that even in small amounts, helium can be dissolved in liquid iron at ultrahigh-pressure conditions, suggesting that there is a reservoir in the Earth’s core. (Taku Tsuchiya)
read more
Transportation of water into the deep Earth by Al-phase D (Dec.1, 2020)
Researchers at Ehime University have recently measured the propagation speed of ultrasonic waves in an aluminum-rich hydrous mineral called Al-phase D at pressure conditions relevant to the Earth’s deep mantle. Their results suggest that seismic shear anomalies observed locally beneath subduction zones may reveal the presence of hydrous minerals in the uppermost lower mantle, which would have important implications for the Earth’s interior because hydrogen affects considerably the physical and chemical properties of mantle minerals. (Steeve Gréaux)
read more
Is the Earth’s inner core oscillating and translating anomalously? (Apr.14, 2020)
A theoretical mineral physics approach based on the ab initio methods was adopted to determine the viscosity of hexagonal, close-packed iron at the extreme pressures and temperatures corresponding to the Earth’s inner core. The results are found to deny geophysical observations of large fluctuations in the inner core rotation rate. The obtained viscosity also rules out inner core translation and provides support that the dynamics of the inner core may be governed by solid-state convection. (Sebastian Ritterbex)
read more
Quantum mechanical simulations of Earth’s lower mantle minerals (Mar.03, 2020)
The theoretical mineral physics group of Ehime University led by Dr. Taku Tsuchiya has developed high-precision computational techniques for studying Earth and planetary materials based on quantum mechanical theory and reported several outcomes for Earth’s lower mantle minerals and high-pressure hydrous phases. Their insights and discoveries clarify the mineralogy of Earth’s lower mantle and new mineral phases stabilized at the deep mantle.(Taku Tsuchiya)
read more
Heat transport property at the lowermost part of the Earth’s mantle (Feb.13, 2020)

Calculated lattice thermal conductivity of MgSiO3 postperovskite (PPv) and bridgmanite (Brg) under the Earth’s lowermost mantle conditions
Lattice thermal conductivities of MgSiO3 bridgmanite and postperovskite (PPv) phases under the Earth’s deepest mantle conditions were determined by quantum mechanical computer simulations. We found a substantial increase in the conductivity associated with the phase change. This indicates that the PPv phase boundary is the boundary not only of the mineralogy but also the thermal conductivity. The effect of anisotropy on the conductivity of PPv in the heat transport properties at the lowermost mantle was also found to be minor.(Haruhiko Dekura)
read more
New aluminium hydroxide stable at extremely high pressure (Dec.11, 2019)
A new hydrous phase, ε-AlOOH, was observed to be stable at pressures above ~200 GPa. The stability of ε-AlOOH at extremely high pressures may affect the modelling results of the internal structure and deep water circulation of some extra-solar planets, such as terrestrial super-Earths, because the hydroxide may store water in these regions.(Masayuki Nishi)
read more
How and when was carbon distributed in the Earth? (Sep.24, 2019)
A magma ocean existing during the core formation is thought to have been highly depleted in carbon due to its high-siderophile (iron loving) behavior. Thus, most of the carbon forming the atmosphere and life on Earth may have been delivered by a carbon-rich embryo after the core formation. However, a new high-pressure experiment has shown that previous studies may have overestimated the amount of carbon partitioning to the core. (Hideharu Kuwahara)
read more
Circulation of water in deep Earth’s interior(Aug.19, 2019)
Phase H is a hydrous mineral that is considered to be an important carrier of water into deep Earth. We determined the dissociation condition of phase H by a theoretical calculation based on quantum mechanics. Phase H decomposes at approximately 60 GPa at 1000 K. This indicates that the transportation of water by phase H may be terminated at a depth of approximately 1,500 km in the middle of the lower mantle. (Jun Tsuchiya)
read more
Why is the Earth’s F/Cl ratio not chondritic?(Jul.1, 2019)
It is believed that terrestrial planets were made from chondrites. However, geochemical observations have shown that the abundance pattern of volatile elements, such as F and Cl in the Earth is inconsistent with chondrites. New high-pressure experiments suggest that F and Cl fractionation during magma ocean crystallization could explain the non-chondritic Earth’s F/Cl ratio. (Hideharu Kuwahara)
read more
Fate of the subducted oceanic crust revealed by laboratory experiments (Jan.31, 2019)
Laboratory experiments at extreme pressures and temperatures lead to precise measurements of the sound velocity of CaSiO3 perovskite which is one of the important constituent minerals in the Earth’s mantle. The measurements suggested the accumulation of the subducted oceanic crust beneath the 660 km discontinuity. (Steeve Gréaux)
read more